At the most basic level, climate changes that cause world surface temperatures to rise are rooted in increased fossil emissions in the atmosphere. Total fossil fuel emissions are a function of key variables, most notably population, per-capita gross domestic product (GDP) and the carbon intensity of an individual unit of GDP.
Understanding these forces and their relationships with each other is critical to measuring the extent of climate change and how we may seek to deal with it. That is the key message conveyed by Dr. John Finnigan, Director of the Australian Commonwealth Scientific and Industrial Research Organisation’s (CSIRO) Centre for Complex Systems Science, who was speaking recently via video-conference to young scientists attending the United Nations University Institute for Sustainability and Peace (UNU-ISP) Postgraduate Courses on Building Resilience to Climate Change.
“We need to ask ourselves what the main drivers of climate change are. And when it comes to climate change, we are really dealing with people, the wealth of people and their aspirations,” Finnigan argues.
To highlight climate change’s socio-economic connection to people, Finnigan and many others cite the Kaya Identity, invented by Japanese scientist Yoichi Kaya. The Kaya Identity is represented by the following equation:
Fossil-fuel emissions = population x per-capita GDP x the carbon intensity of GDP.
Since the 1980s, as this graph below shows clearly, increasing world population and wealth growth have increased global emissions significantly. At the same time, the decreasing carbon intensity of GDP (that is, less carbon is being emitted to produce each unit of GDP) means that fewer emissions are required to produce wealth and lift people out of poverty.

Raupach et al. (2007) PNAS Updated with IEA data to 2006
Four drivers of the climate system
Finnigan explains that at the heart of the climate system are four key drivers, the growth of which will determine the extent to which future predictions of climate change turn out to be true over the new few decades. These four drivers are:
1) population
2) aspiration
3) connectivity
4) biogeochemical change
It is generally assumed that the first of these, population, will continue to rise globally to around 9 billion people in 2050. And while the earth’s population is rising fast, is it rising at a decreasing rate. These trends are explained by the idea of “demographic transition”, where people start to live longer and have fewer children. It is commonly accepted that demographic transition will continue to take place in developing countries as it has done in developed countries.
Related to this, there is a proven correlation between higher GDP and lower fertility rates, even though the causal relationships may be contested. In real numbers, population replacement levels of 2.1 children per woman occur when per capita GDP levels are around US $5000.
At the same, time, the world has passed the point where 50% of the global population lives in urban areas, leading to a reliance on outlying and often faraway regions to harvest and transport power, food and water into cities, as well as to accommodate waste removal.
The second key variable is that of aspirational change, which has mainly occurred since World War II. Broadly speaking much of the wealth lies with a minority of the global population that is mainly concentrated in the global North: North America, Western Europe and parts of the Pacific rim. However, in the last 20 years, in particular since the fall of the Berlin Wall and the opening up of China, wealth generation has shifted eastwards and southwards to the BRICS (Brazil, Russia, India, China, South Africa) countries and beyond.
Connectivity is the third and least understood variable. It has increased exponentially since WWII in the same way that population growth and wealth growth have. More on connectivity in a moment.
The fourth and final driver is that of biogeochemical change. This includes negative trends in key biophysical indicators such as levels of atmospheric concentration of greenhouse gases (GHGs), ozone depletion and biodiversity loss — again, particularly since the 1950s. When it comes to making future projections, it is critical to recognize how many of these biogeochemical changes are locked-in for the next 20 or 30 years or even longer, no matter what is done now to slow down their impact.
“We are committed to some level of global warming even if we stop emitting carbon today. Ecosystems continue to change for quite some time after you impact them,” Finnigan explains.
The one positive note here is that institutional and technological improvements are not yet determined and some positive changes to the system can still take place. Many researchers, policy-makers and business people are placing their hopes in post-fossil fuel techno-fixes such as genetically modified crops and carbon capture and storage technology. Even still, Finnigan issues a further word of caution:
“We are now impacting ecosystems even faster as they continue to adjust to our past impacts. It is very difficult to know what the consequences of this will be.”
The connectivity paradox
Understanding driver number three — connectivity — can help us put the climate change and other challenges into perspective, according to Finnigan. As his following 5-graph sequence illustrates, the dynamics of connectivity can be understood by thinking of systems as a collection of ‘nodes’ and ‘links’ connecting these nodes.
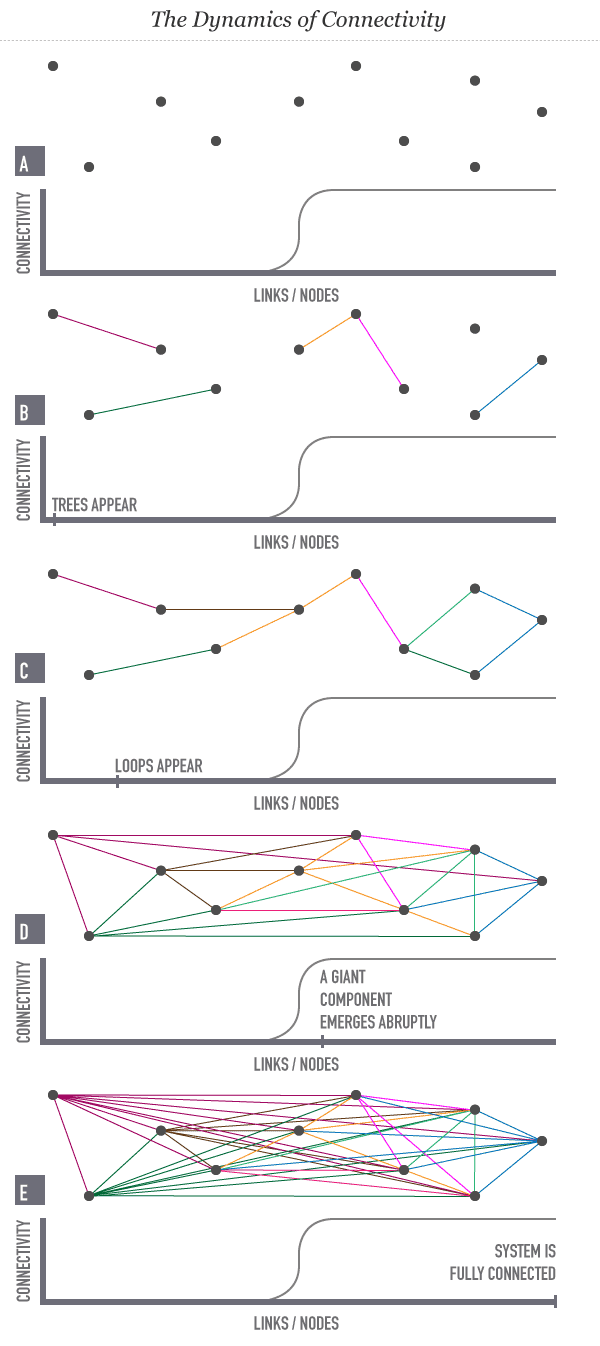
As applied to the world economic system throughout history and today, nodes represent cities, towns or even companies, and links represent trade or communication routes. We can think of the first few graph sequences (A-C) as representing the progression from unconnected early civilisations to regional empires, and then from colonialisation to the industrial revolution. As globalisation has intensified, particularly since World War II (D), more and more links are added connecting more and more nodes to each other, until the point today (E) where the world trade network is fully connected.
What is interesting and perhaps unexpected here, as the third graph in the sequence illustrates, is that connectivity between the nodes jumps suddenly at around the point where you have half as many links as nodes. Eventually, connectivity expands to the point where the system is virtually fully connected, in the same way as the global economy.
“Today we have a fully connected world system. What mathematicians mean by that is that we can get at any one of nodes from almost any other along the links,” Finnigan explains.
In relation to the world food trade that has been under scrutiny of late, high connectivity enables supply of essential agricultural inputs such as fuel and fertilizer, thereby ensuring that much of the world’s population remains nourished. At first glance, global interdependence appears beneficial as countries lacking in crucial commodities — such as oil and staple food crops like wheat, maize and rice — can easily import them.
“The current global trade in food is absolutely essential to feed the global population and maintain supplies around the world,” Finnigan points out.
Yet, as we have witnessed, particularly since the global financial crisis, a highly connected system is extremely vulnerable to small shocks that can spur on “unpredictable feedbacks in the system”. Recent uprisings in North Africa have continued to nudge the world oil price higher and that has flow-on effects for the already record-high food price index.
The structure of the world trade network is inherently unstable in two different and complementary ways. First, its high connectivity means that shocks to the system, such as a rise in oil prices or converting a part of the world’s maize production to biofuels, can propagate and cause large unexpected oscillations in price and availability. An example of this was the food price spike in 2008 when a combination of energy price rises, crop diversions for biofuel use and market speculation intersected, shocking the system.
Secondly, it is structured in such a way that much world trade flows through a few large nodes or hubs or via links that carry a high volume of traffic. According to Finnigan, just 10% of the links carry more than half of the total flows within the system. When a critical link is cut or the flow through a hub is blocked, then large slices of the trade network can be affected.
The first figure below represents the world trade network where unstable hubs (imagine London, New York and Tokyo) are closely linked and therefore the network is vulnerable. Alternatively, the second figure depicts stable hubs that are separated and thus more resilient to shocks that may affect other hubs.
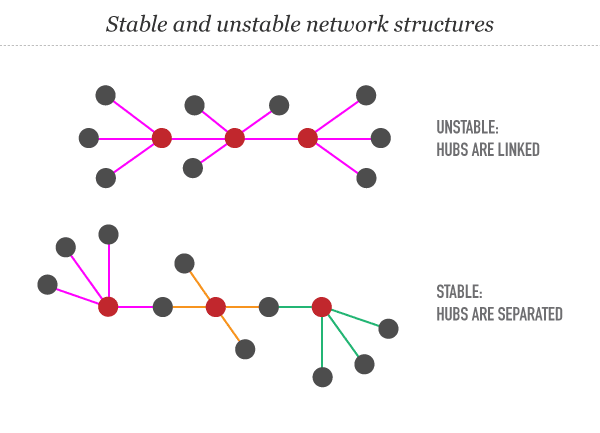
As an example of a critical link, Finnigan cites the almost annual spat between Russia, suppliers of 25% of the Europe Union’s gas, and the Ukraine, which transports gas between Russia and its European customers. What happens between Russia and the Ukraine can cause, almost overnight, an exponential cost increase in energy for millions of people living thousands of kilometres away.
This situation where connectivity can both prevent and exacerbate global problems leads to a “paradox of connectivity”. On the positive side, with so many links between major nodes, one might expect that if some of these break down, then traffic can simply be diverted through other nodes as the system as a whole readjusts itself. On the negative side, the structure of the network means that unexpected oscillations in food price and availability are inevitable and these affect the world’s poor to a disproportionate degree as so much of their income is spent on food.
Designing better networks
Moving beyond food, capping and eventually reducing population requires an increase in wealth for that majority of the world’s population that currently shares only a small part of the world’s economic productivity. This requires economic growth but also requires decoupling economic activity from negative impacts on the world’s vital systems. This will require a much deeper understanding of how the world’s natural economic and social systems interact.
As a complex systems scientist, Finnigan feels strongly about the importance of interdisciplinary macro level analysis and its ability to help us plan for and respond to complex global problems. For this, much more work needs to be done to draw the fields of economics and social science into a partnership with natural science for a more integrated way of thinking about global dynamics.
The problems we have identified with instability of the world trade network applies with even more force when we contemplate world networks of financial and information flows. Many of the critical links and nodes in these systems involve human action both by individuals and groups. The increase in size and complexity of the world’s networks — due to continued global population increases, urbanization and economic growth — is guaranteed to lead to serious problems unless we are aware of how network dynamics underpin our modern world.
To confer resilience against unexpected future shocks we need to design systems that trade off some short-term economic efficiency for long-term stability and the ability to ‘fail safely’. Many leading polymaths such as Dennis Meadows, point out that a blind drive for economic efficiency, narrowly defined, can led to reduced resilience and greater risks of shortages in food.
But does that mean we simply eliminate trade and move towards local solutions to mitigate climate change and ensure food security?
Finnigan reiterated that solutions should reflect the fact that a connected world can continue to lift people out of poverty and slow population growths. Further still, withdrawing from a globalised world is not an option for many communities that rely on international trade and food supply, despite systemic instability of supply. So where do we go from here?
“We can definitely do things better. If we recognize the role of network dynamics, we can design national and global institutions and regulations that take advantage of networks while mitigating their downsides. It is possible to have large interconnected networks that are capable of avoiding large oscillations, but you have to design them that way to begin with,” Finnigan says.